What is PTP (Precision Time Protocol)? Understanding IEEE 1588 and Grandmaster Clocks
Precision Time Protocol (PTP), defined by IEEE 1588, is a clock synchronisation protocol. It is used in systems that need very accurate timing, often within sub-microseconds. From financial transactions to power system automation and military networks. PTP ensures that all devices within a network are operating in perfect time alignment.
In this guide, we’ll explore what PTP is, how it works, the role of Grandmaster Clocks. We will also discuss how EON Instrumentation provides PTP solutions that are very reliable and accurate.
🕓 What is PTP?
PTP (Precision Time Protocol) is a network-based time synchronisation standard. That enables clocks in distributed systems to align within nanoseconds of a central time source. Unlike NTP (Network Time Protocol), which offers millisecond-level precision. PTP compensates for network latency and jitter, delivering the accuracy needed for time-sensitive operations.
Applications of PTP include:
- Financial systems requiring accurate transaction timestamping
- Substation automation and circuit breaker sequencing in power grids
- Telecommunications and broadcasting synchronisation
- Aerospace and defence systems with mission-critical timing
🧭 The Role of the Grandmaster Clock (GMC)
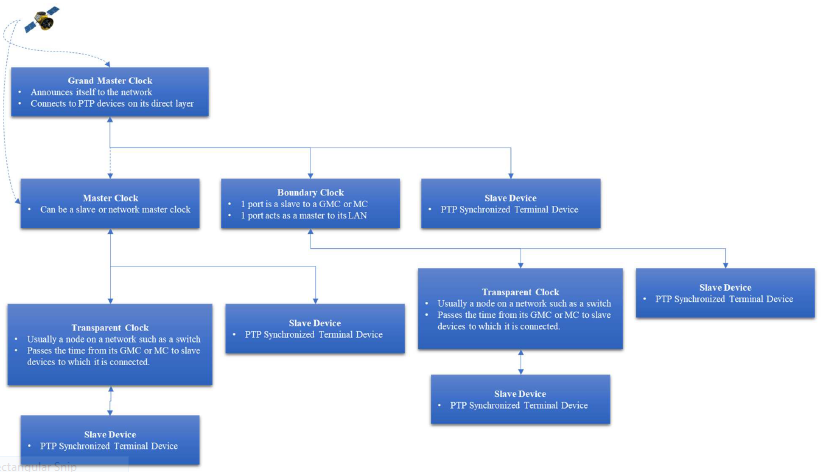
At the heart of every PTP network is the Grandmaster Clock (GMC). The system’s most accurate and authoritative time source. The Grandmaster is typically synchronised to a GPS or atomic clock, qualifying it as a Stratum 1 device.
This clock distributes time information across the network to:
- Master Clocks (MCs) – Intermediate timing devices
- Boundary Clocks (BCs) – Synchronise devices across network segments
- Transparent Clocks (TCs) – Compensate for network switch latency
- Slave Devices – End devices that receive and align with distributed time
The network dynamically uses the Best Master Clock Algorithm (BMCA) to select the optimal Grandmaster when multiple candidates are available.
🔁 Syncing Over a Network: How PTP Works
PTP relies on frequent sync messages passed between clocks. However, as data packets traverse routers, switches, and other network equipment, they experience variable delays (latency).
To overcome this, PTP uses several key techniques:
- Transparent Clocks adjust timing based on known network delays
- Boundary Clocks isolate timing domains to reduce drift
- Hardware Timestamping captures send/receive times at the physical layer
- Oscillator Disciplining keeps clocks aligned even during temporary outages
These mechanisms ensure time synchronisation remains consistent across the entire network—even over complex and congested infrastructure.
📉 Clock Drift and Stability
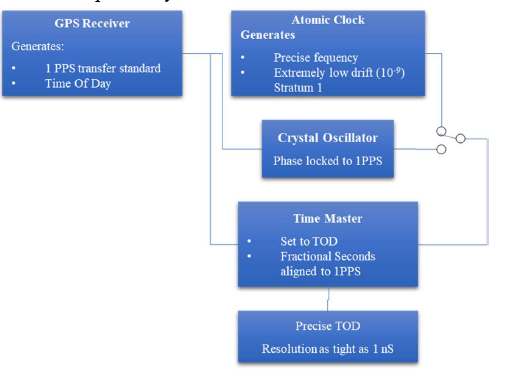
All clocks drift due to environmental factors like temperature or component aging. In a PTP system, time masters use disciplined oscillators and intelligent correction techniques. This minimises drift and maintain time integrity.
🛠️ EON Instrumentation’s PTP Solutions
EON Instrumentation offers various PTP-enabled timing devices. These devices are made for challenging environments in industries like industrial, aerospace, and defence. Their products are engineered for:
- Minimal clock drift
- Seamless integration with legacy systems
- Compatibility with IRIG B, SMPTE, and PTP IEEE 1588-2008 standards
- Resilience during power loss or signal disruption
EON’s systems are ideal for customers upgrading from older timing standards or deploying precise synchronisation over Ethernet networks. To learn more about IRIG timecode consider reading this article.
🌏 Available in Australia and New Zealand
Metromatics proudly represents EON Instrumentation in Australia and New Zealand. Offering local expertise and support for PTP, IRIG B, and SMPTE timing systems. Whether you’re upgrading infrastructure or designing a new network, we can help deliver precision and reliability.
👉 Contact us to discuss your application or request a quote.